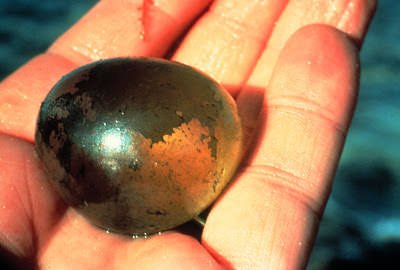
The simplest of lifeforms are often the most fascinating. In high school biology, we learn that the fundamental state of all living things is the cell, and students spend a lot of time pouring over cartoons and blurry microscope images of a cell’s function and anatomy. Cells are microscopic, so it can be hard to visualize the wide array of biological processes—diffusion, osmosis, reproduction, etc—and the scale on which they occur. It would be nice if a cell was large enough to hold in the palm of a hand, allowing us to relate the inner workings of a cell to the scale of our world. Such a thing exists but we must dive into the ocean to find it. Ubiquitously, upon the seafloor, we find the algae Valonia ventricosa, or “sailor’s eyeballs”, which provide a lot of macroscopic insight to certain aspects of the cell that we normally relate to on a microscopic level.
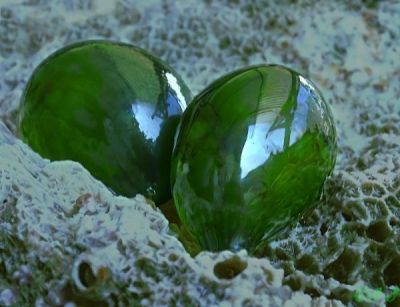
At first glance, Valonia ventricosa looks like a shiny, featureless blob. On a second glance, it’s…still a blob. There is really nothing exciting visually about these organisms and this is fine because the important stuff lies within. Its anatomy is simple: a membrane that encapsulates chloroplasts (for photosynthesis because it is an algae), some rhizoids, multiple nuclei containing DNA and other functional parts, and a large central vacuole (effectively a storage unit for energy). Simple anatomy explains its existence as one of the largest single-celled organisms, as opposed to multicellular organisms like a starfish (or a human, of course), which have a vast differentiation of cell types performing specific functions for the organism’s survival. Bacteria is the common example of a single-celled organism but it is invisible to the naked eye and therefore not always accessible to the imagination, yet Valonia ventricosa populate the ocean like crumbs on the kitchen floor.
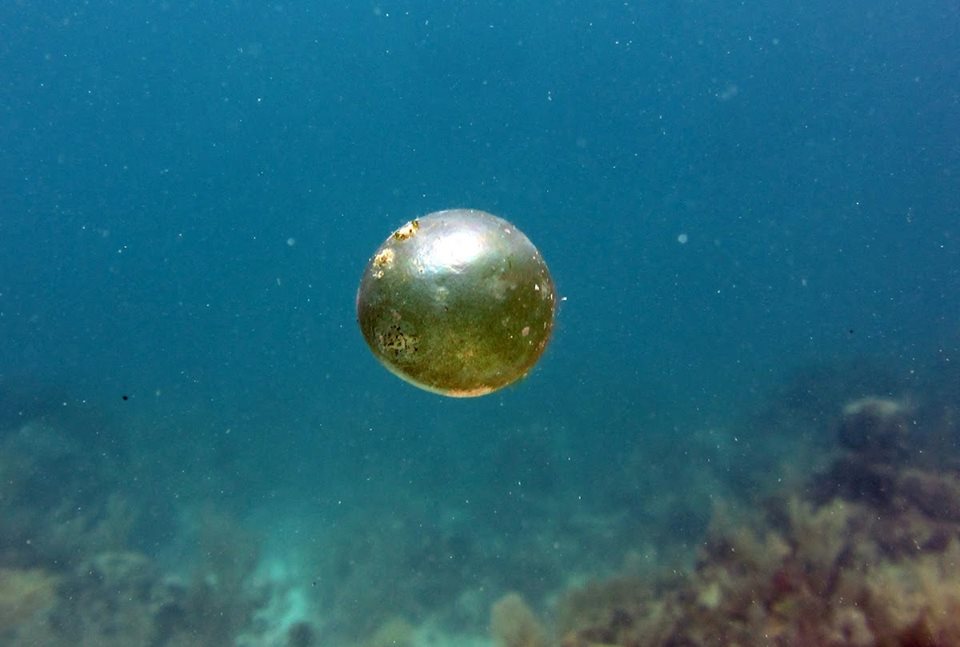
Valonia ventricosa’s large, single cell-iness has made the organism a scientific focus on how cell membranes function. As you may or may not know, the membranes of cells are crucial because they allow nutrients, chemical signals, and genetic material to pass into and out of a cell into a substrate (like blood or water) or into another cell. Membranes also keep the important genetic material of the cell differentiated from other locations within a cell and provides a degree of protection from the chaotic exterior of the outside world. When Valonia ventricosa was determined to truly be just a large, single cell, the first question was then: Does it still act like its smaller, single cell counterparts? The answer was yes. Microscopic plant cells rely on the transfer of water and water-soluble molecules to cross membranes. Because Valonia ventricosa has some similarities (but is not identical) to the anatomy of a plant cell, scientists are interested in the same transfer of water-based materials across the membrane, specifically the turgor pressure of its membranes.
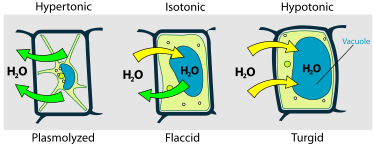
Simply put, turgidity is a measure of the fluid pressure of a membrane against the cell wall that holds it, and when water meets a membrane, the turgidity of a cell’s membrane must increase or decrease according to the needs of the cell in that moment. Membranes, in general, have turgidity because the membrane itself is semi-permeable, allowing some materials, like water, to pass through while blocking other materials, like certain molecules and ions. In the case of Valonia ventricosa, a study from the Journal of Membrane Biology has shown that the organism may be actively transporting potassium ions from the exterior salty water of the ocean in much the same way as the microscopic cells do within the salty-ish fluid of our own bodies. Evidence for this in Valonia ventricosa is demonstrated by measuring a relationship between the organism’s interior potential difference (from ions in salt water) and the amount of turgidity/fluid stress on its membranes. Typically, microscopic cells deal with much smaller fluid forces upon their membranes, so when Valonia ventricosa performs similar functions but in response to forces exponentially larger than those occurring in small cells, it creates a lot of curiosity on the exact nature of membrane transport.
Much like how it is appealing to see tigers and lions exhibit the same behaviors as a house cat, it is very satisfying to me that we can physically see a giant cell perform fundamental and typically microscopic functions with our own eyes. I suppose we’re not really seeing the function of the cell but now it feels more accessible in my mind when thinking about Valonia ventricosa. Maybe you feel the same way, or maybe not. But nonetheless, the mechanisms of membrane transport create a vast and complex field, and studies of the organism can easier place into perspective the intriguing nature of the invisible cells that work everyday in our own bodies to keep us alive and well.